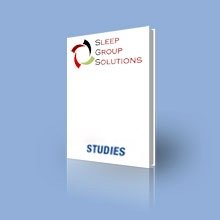
Mandibular Advancement for Obstructive Sleep Apnea Relating Outcomes to Anatomy
Source: https://jamanetwork.com/journals/jamaotolaryngology/fullarticle/1775607
Mandibular Advancement for Obstructive Sleep Apnea Relating Outcomes to Anatomy
Importance This study provides insight into the response and cure rates of oral appliances (OAs) in patients with primary retropalatal, retroglossal, or retroepiglottic obstruction, as well as the effect of minimal cross-sectional area on the overall decrease in the apnea-hypopnea index (AHI) across all anatomical locations of collapse.
Objective To examine the role of regional upper airway obstruction measured with acoustic pharyngometry as a determinant of OA success.
Design, Setting, and Participants This retrospective case-series included patients with obstructive sleep apnea-hypopnea syndrome at a tertiary care center.
Interventions Patients were fitted with a custom OA between July 1, 2011, and January 1, 2012.
Main Outcomes and Measures Regions of maximal upper airway collapse were determined on acoustic pharyngometry: retropalatal, retroglossal, or retroepiglottic. Apnea-hypopnea index improvement at titration polysomnography was assessed against regional collapse.
Results Seventy-five patients (56 [75%] men; mean [SD] age, 49.0 [13.6] years; mean body mass index [calculated as weight in kilograms divided by height in meters squared], 29.4 [5.2]; and mean AHI, 30.6 [20.0]) were assessed, and data were grouped on the basis of region of maximal collapse at pharyngometry (retropalatal in 29 patients, retroglossal in 28, and retroepiglottic in 18). The overall reduction in AHI at OA titration showed no significant difference between groups. There was no significant difference in the response rate to treatment, defined as more than 50% AHI reduction plus an AHI of less than 20 (response rate, 69% for retropalatal, 75% for retroglossal, and 83% for retroepiglottic collapse; P = .55) or the cure rate, defined as an AHI of less than 5 (cure rate, 52% for retropalatal, 43% for retroglossal, and 72% for retroepiglottic collapse; P = .15). The correlation between minimal cross-sectional area and response trended toward significance (r = 0.20; range −0.03 to 0.41; P < .10).
Conclusions and Relevance Oral appliance therapy achieves reasonable response and cure rates in patients with primary retropalatal, retroglossal, or retroepiglottic obstruction at the time of initial titration polysomnography. However, success is not predicted by identification of the region of maximal upper airway collapse measured with acoustic pharyngometry.
Obstructive sleep apnea is caused by an obstruction of the upper airway, either from soft-tissue collapse or decreased patency. The goal of treatment, therefore, focuses on decreasing the propensity of the airway to collapse and increasing the airway cross-sectional area.
In 2009, the American Academy of Sleep Medicine recommended 2 forms of treatment for mild to moderate obstructive sleep apnea–hypopnea syndrome (OSAHS): continuous positive airway pressure (CPAP) or mandibular advancement devices (MADs), alternatively called oral appliances (OAs).1 To date, the US Food and Drug Administration has approved multiple different OAs for use as a first-line treatment for mild to moderate OSAHS.1,2 Studies have shown that there is no statistically relevant difference in the success rates of MADs vs CPAP for mild to moderate OSAHS when both devices are titrated correctly.3
Multiple techniques, including cephalometric analysis and imaging combined with computational fluid dynamics, have been researched in an effort to clarify the exact method of action and establish a way to predict the treatment success of MADs.4,5 These studies have shown mixed results with respect to predicting MAD treatment success in patients.
Pharyngometry is a less effectual technique that is also being used in the evaluation and treatment of OSAHS. This method emits sound waves through a mouthpiece and records the wave amplitude as it reflects back off the soft-tissue structures of the oropharynx and hypopharynx. The data are then depicted as a waveform that can be used to identify and classify the site of maximal airway collapse. These specific acoustic airway measurements have been previously shown to correlate well with computed tomographic measurements in awake patients with spontaneous breathing and have been shown to have excellent test-retest reliability and validity.6,7
The purpose of this study was to identify the anatomical site of maximal collapse through the use of pharyngometry and to determine whether the site of collapse can be used to predict the success of OSAHS treatment using custom MADs. The 3 anatomical divisions used to classify obstruction were primary retropalatal, retroglossal, and retroepiglottic. We compared the pretreatment polysomnography apnea-hypopnea index (AHI) with the titration polysomnography AHI and correlate the improvement in AHI with the anatomical region of maximal collapse. We hypothesized that there would be a strong correlation between maximal retroglossal collapse, as determined by pharyngometry, and improvement in the AHI with use of the custom OA. The retroglossal site would seem the most likely to benefit from the use of MADs. Currently, there are limited data to support this hypothesis based on anatomical relationships of the pharynx.
This study was an observational retrospective review. Institutional review board approval (Advocate Health Care) was obtained before the study was begun. Medical records were reviewed from consecutive adult patients (aged >18 years) with a diagnosis of OSAHS who presented for custom OA fitting between July 1, 2011, and January 1, 2012 at an outpatient site in Chicago, Illinois (Advanced Center for Specialty Care). In all patients a trial of CPAP had previously failed.
Data collected included patient demographic information (age and sex) and physical examination findings (body mass index [BMI], calculated as weight in kilograms divided by height in meters squared; Friedman tongue position; and tonsil size), pharyngometric data at both tidal and residual volume (maximal collapse location, minimal cross-sectional area, and mean airway area), and initial and titration polysomnographic data (AHI and minimal oxygen saturation). All initial polysomnographic studies were performed within 3 months before device fitting, and all were performed at the same facility and analyzed by the same scorers and the same sleep physician.
Inclusion criteria for review of data were (1) age of at least 18 years, (2) diagnosis of OSAHS, (3) AHI of at least 5 at pretreatment polysomnography, (4) failure of CPAP, and (5) completion of polysomnography. Patients with temporomandibular joint disorders or severe nasal obstruction were excluded.
The TAP 3 (Thornton Adjustable Positioner; Airway Management, Inc) is a custom-made 2-piece titratable MAD, as seen in Figure 1, that fits over the upper and lower dental arches. It is connected by one of several interchangeable hooks that allow for differences in bite class, and it has a screw that allows for adjustment of mandibular protrusion with the device in or out of the mouth.
Alginate impressions and protrusive bite registration are performed. The device is then fabricated in an off-site dental laboratory. Fitting and adjustment of the device are performed by the dentist at a second appointment 14 to 21 days after the initial visit. The device is then optimized during titration polysomnography, which is scheduled by the patient immediately after the fitting. The titration of the device is comparable to CPAP titration. If the sleep technician sees apnea or hypopnea during the night, the device is titrated (by adjusting the mandibular protrusion) until a normal AHI is reached or until the patient starts to feel discomfort. At the point of discomfort, the device is titrated down to achieve a comfortable protrusion with optimal titration.
The Eccovision Acoustic Pharyngometer (Sleep Group Solutions) was used for pharyngometric measurements. This device uses a wave tube with an attached mouthpiece on which the patient bites down. The mouthpiece consists of a bite plate for the teeth, as well as a flange that is placed between the anterior tooth surface and posterior lip mucosa to provide an acoustic seal. The nasal passages are occluded to prevent any sound waves from escaping. Sound waves are emitted from the wave tube, travel to the airway tissues, and reflect back to a sensor in the wave tube. The acoustic wave amplitude and associated timing is recorded, transmitted to a computer where the data are analyzed, and translated into a pharyngogram (Figure 2). Acoustic measurements were performed at both lung tidal volume and expiratory residual volume to mimic the sleeping/apneic state as closely as possible.
The pharyngogram provides a graphic representation of the upper airway anatomy. The x-axis corresponds to the distance from the teeth, and the cross-sectional area (in square centimeters) is denoted on the y-axis. The amplitudes of the returning sound waves are converted into data points, which are then plotted on a graph in respect to the x- and y-axes. The resulting line graph correlates to anatomical landmarks and cavities: the oral cavity, oropharyngeal junction, oropharynx, epiglottis, glottis, and hypopharynx. The locations of these anatomical sites on the pharyngogram have been previously identified and validated by other studies.8
Using the previously validated normal pharyngogram and the associated known anatomical landmarks as a comparison, we were able to identify the anatomical location of maximal collapse on each of the study pharyngograms. The collapse location was then classified into 1 of 3 categories: retropalatal, retroglossal, or retroepiglottic. Figures 3, 4, and 5 are pharyngometric graphic representations of each category of obstruction.
The first use of the MAD was at titration polysomnography, which was scheduled by the patient immediately after fitting. Parameters (AHI and minimal oxygen saturation as measured by pulse oximetry) recorded at the optimum degree of mandibular protrusion were used for data analysis. Response was defined objectively as a decrease in AHI by at least 50% compared with baseline and an AHI of less than 20. This was based on the commonly accepted cutoff for defining surgical success. Cure was defined as achievement of an AHI of less than 5.
The only criterion for exclusion from final analysis was incomplete or unavailable titration polysomnographic data.
Data from 75 patients who met all the listed criteria and had complete polysomnographic records were analyzed. The 75 patients were divided into 3 groups based on the site of maximal collapse. These groups were analyzed for baseline characteristics: age, sex, BMI, and pretreatment AHI. Pretreatment and posttreatment AHI, change in oxygen saturation as measured by pulse oximetry, site of maximal collapse, and minimal cross-sectional area at both tidal and residual volumes were compared within groups. Pretreatment AHI, change in AHI, and response/cure rates were compared between groups.
All continuous data are presented as means (SDs). Paired t tests were used to compare continuous variables within groups before and after treatment, 2-tailed independent t tests were used to compare continuous variables between groups, and χ2 analysis was used to test the association of categorical variables. Differences were considered statistically significant at P < .05.
Seventy-five patients (56 [75%] men; mean [SD] age, 49.0 [13.6] years; mean BMI, 29.4 [5.2]; and mean AHI, 30.6 [20.0]) were assessed and data were grouped according to the region of pharyngometric maximal collapse at respiratory residual volume. The residual volume measurements used to categorize patients are detailed in Table 1. The sample sizes for the respective groups were 29 patients for retropalatal, 28 for retroglossal, and 18 for retroepiglottic collapse. Table 2 elaborates on the specific characteristics of each group with regard to mean age, BMI, sex, and pretreatment AHI. The difference in proportion of men to women in the various groups was not significant (χ2 = 0.96).
When we correlated the minimal cross-sectional area or the location of the minimal cross-sectional area with the change in AHI for all patients, we noted no significant correlation (r = 0.08 and −0.05, respectively). The minimal cross-sectional area location and pretitration AHI over all anatomical groups also showed no correlation (r = 0.00013; P = .99). The reduction in AHI at MAD titration showed no significant difference between groups. In addition, there was no significant difference in the rate of response to treatment (defined as >50% AHI reduction plus an AHI of <20; response rate, 69% for retropalatal, 75% for retroglossal, and 83% for retroepiglottic collapse; P = .55) or the cure rate (defined as an AHI of <5; cure rate, 52% for retropalatal, 43% for retroglossal, and 72% for retroepiglottic collapse; P = .15), as shown in Table 3.
One of the challenges of treating OSAHS is our lack of ability to understand a patient’s unique airway anatomy and how that anatomy contributes to the evolution of OSAHS. In addition, the field lacks an effective way to use the available anatomical information and translate it into a systematic method for predicting specific treatment success. This can lead to ineffective, costly, and sometimes irreversible effects that negatively affect patients.
Cephalometry, the long-accepted standard for structural evaluation of OSAHS, allows only a 2-dimensional lateral representation of the airway. The dynamic nature of the upper airway throughout the respiration cycle, and the fact that it is a 3-dimensional soft-tissue structure hinder the ability of cephalometry to demonstrate the severity of OSAHS or accurately predict treatment success.9 Although studies have previously linked specific cephalometric values to MAD success, such as overjet and vertical height of the hyoid bone, additional prospective validation studies are needed to determine the validity of these results and their applicability to clinical practice.10 Moreover, specific cephalometric measurements more accurately predict postsurgical success.11 However, more research is still needed to improve our ability to define the relationships between anatomy and the success of specific treatments.
Computed tomographic evaluation, with or without computational fluid dynamic analysis, has been used to create a digital 3-dimensional rendition of the airway in an attempt to better link airway anatomy to treatment success.12 The findings of this technique, when used in conjunction with BMI, correlate with OSAHS severity.12 However, although understanding the severity of the disease helps determine which treatment options to consider, it does not allow us to predict the success of treatment with an OA.
Pharyngometry allows an inexpensive, rapid, in-office method of obtaining a graphic and numeric representation of the upper airway dimensions, allowing indirect evaluation of the cross-sectional area of the airway. This method has been previously shown to correlate with computed tomographic evaluation.7 To our knowledge, the present study is the first to use pharyngometry to predict which patients will have success with MAD treatment based on the anatomical region of airway collapse. We hypothesized that patients with retroglossal collapse would have the greatest improvement in AHI. This hypothesis was founded on the anatomical relationships of the pharynx as well as the way in which the OA affects the soft tissues of the airway, most noticeably its direct effect on the retropalatal and retroglossal regions of the oropharynx.13
Contrary to our hypothesis, success with OA therapy was not predicted by the region of maximal upper airway collapse. Rather, MAD therapy achieved reasonable response and cure rates in patients with primary retropalatal, retroglossal, or retroepiglottic obstruction as measured by initial titration polysomnography. The only factor that could be noteworthy at demonstrating a correlation (that was not seen across all groups) was a trend toward significance (r = 0.20 [range, −0.03 to 0.41]; P < .10) when correlating minimal cross-sectional area location and response to treatment.
Possible limitations of this study include the method by which the pharyngometric measurements were taken. Pharyngometric findings have been proved to correlate accurately with patients’ individual physiology through comparisons with computed tomographic images of awake patients breathing spontaneously while in the supine position; in our study, however, pharyngometry was performed with patients seated.7 The change in measurement position could change the anatomy of the airway or interfere with the ability of the pharyngometer to provide an accurate evaluation of the airway. Either of these possibilities could cause the anatomical location of collapse to be misidentified and a patient to be classified incorrectly. However, because such a statistically significant improvement in AHI was seen across the patient population, any errors that may have been induced can be concluded not to have changed the underlying observation of the study, which was that the use of MADs led to an improvement in AHI regardless of anatomy. Future studies could avoid this limitation by performing measurements with patients in the supine position.
The primary clinical shortcoming of this study is the relative obscurity of pharyngometry in otolaryngology practices. Although pharyngometry is used by dentists to aid in titrating MADs for OSAHS, it has not yet become commonplace in ear, nose, and throat practices. However, the success of MADs, as shown by our study, does not correlate with the anatomical location of airway collapse. It is therefore reasonable to suggest that any patient who meets the current clinical criteria for a MAD should be considered for treatment with a custom-fit device that is titrated using polysomnography.
In conclusion, success with OA therapy is not predicted by identification of the region of maximal upper airway collapse as measured by acoustic pharyngometry in the sitting position. Therapy with an OA achieves reasonable response and cure rates in patients with primary retropalatal, retroglossal, or retroepiglottic obstruction at the time of initial titration polysomnography. Minimal cross-sectional area also had no effect on the overall decrease in AHI across all collapse sites. These results show that any patient who currently fits the clinical criteria for MAD therapy, and in whom a CPAP trial has failed, could benefit from a custom-fit titratable device for OSAHS treatment.
Submitted for Publication: January 2, 2013; final revision received August 21, 2013; accepted September 18, 2013.
Corresponding Author: Michael Friedman, MD, Chicago ENT: An Advanced Center for Specialty Care, Advocate Illinois Masonic Medical Center, 30 N Michigan Ave, Ste 1107, Chicago, IL 60602 (mfriedmanmd@gmail.com).
Published Online: November 21, 2013. doi:10.1001/jamaoto.2013.5746.
Author Contributions: Drs Friedman and Shnowske had full access to all of the data in the study and take responsibility for the integrity of the data and the accuracy of the data analysis.
Study concept and design: Friedman, Hamilton, Samuelson, Hirsch.
Acquisition of data: Hamilton, Samuelson, Pott, Yalamanchali.
Analysis and interpretation: Friedman, Shnowske, Hamilton, Samuelson, Pott.
Drafting of the manuscript: Friedman, Shnowske, Hamilton, Samuelson, Pott.
Critical revision of the manuscript for important intellectual content: All authors.
Statistical analysis: Friedman, Shnowske, Hamilton, Samuelson, Pott, Yalamanchali.
Administrative, technical, and material support: Friedman, Hamilton, Samuelson, Hirsch, Pott.
Supervision: Friedman.
Conflict of Interest Disclosures: Dr Samuelson reports being a consultant to the Restech and Epitopoietic Research Corporation. Dr Friedman reports receiving a research grant from Ventus Medical, Inc (now Theravent, Inc). No other disclosures were reported.
Funding/Support: This study was funded in its entirety by the principal investigator (Dr Friedman).
Previous Presentation: Presented in part at the American Academy of Otolaryngology–Head and Neck Surgery Foundation 2012 Annual Meeting and OTO EXPO; September 9-12, 2012; Washington, DC.
Additional Contributions: We thank Mary E. Lundgren, PhD, for assistance with the revision of this manuscript and all support provided, Dr Hirsch for Dental Sleep Medicine service coordination, and the clinical and administrative staff of the Advanced Center for Specialty Care.